Introduction
Few elements are as important and useful as iron among the wide range of elements that make up the periodic table. Iron has been essential in forming human history and technological advancement from prehistoric societies to contemporary industries. We will examine the qualities, applications, and eternal significance of iron in many facets of our existence as we delve into this blog.
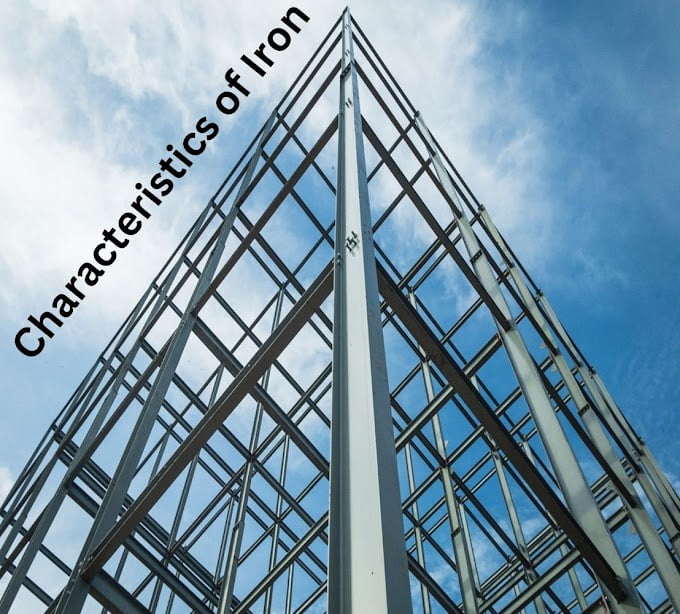
Chemical and Physical Properties
- Density and Melting Point: In other words, iron can be identified as a relatively heavy metal with a density close to 7.87 g / cm3. It holds a relatively high melting point- (1,538 degrees Celsius) which make it ready for high-temperature applications.
- Magnetic Properties: Introducing a field, the iron is ferromagnetic i.e it is characterized by the strong magnetic outcome. These unique features enable iron to be used for the creation of transformers, motors, electrical industries, and other magnet-based technologies.
- Corrosion Resistance: While pure iron is bad at being rust proof when the part of it is there is moisture and oxygen, there is the group of iron alloy, called stainless steel, which has the properties of high corrosion resistance because chromium and the others are added as the alloy elements.
- Ductility and Malleability: As it often the case, iron exhibits ductility and mallability, i.e. it can be pulled into wires and bounded into thin plates without braking them. This characteristic of the range of iron alloys endows them with such an incredible adaptability and malleability that they are especially fit for the performance of different manufacturing procedures.
Cooling curve for pure iron
Iron may exist in several allotropic forms in the solid state at different temperatures.The transformation of iron from one allotropic form to another is accompanied either by the evolution of heat or absorption of heat. Fig. 1 shows the cooling curve of pure molten iron (melting point ⋍ 1537 ° C) plotted in time v’s temperature coordinates.
The first horizontal step appears on this curve at a temperature of 1537° C. It indicates that heat is evolved and that iron passes from the liquid to solid state. The mixture consists of liquid plus delta (δ)- iron solid solution. Delta iron has body centred cubic (b.c.c.) crystal lattice. The second temperature effect occurs at 1392° C and delta iron gets transformed into gamma (γ)- iron, which has a face centered cubic (f.c.c.) lattice.
Next step is at 910° C where γ -iron is transformed into beta (β)- iron with b.c.c. lattice. The fourth step is observed at 768° C, where β - iron is transformed into alpha (α) -iron with (b.c.c.) lattice. α - iron acquires pronounced ferro-magnetic properties.
There are actually two allotropic forms of iron : α - iron and γ - iron as follows : –
- δ - iron (b.c.c.) : 1392° C – 1537° C
- γ - iron (f.c.c.) : 910° C – 1392° C
- β - iron (b.c.c.) : 768° C – 910° C
- α - iron (b.c.c.) : < 768° C
β-iron may be regarded as a paramagnetic state of α - iron.
Critical Points. On the cooling curve (or on the heating curve), the points where structural changes occur, are known as “critical points”. In Fig 1, the critical point of the α⇌γ.
transformation at 910° C is denoted by Ar3 (in cooling) and by Ac3 (in heating). The critical point of the γ⇌α transformation at 1392° C is denoted by Ar4 (in cooling) and by Ac4 (in heating). Again, the critical point (768° C) corresponding to magnetic transformation of α = iron is denoted by Ar2.Letter A denotes arrest, r for cooling and c for heating. The significance of “arrest” lies in the fact that structural changes take place at constant temperature.
Absolutely pure iron is very difficult to obtain. But, in this state, it is a soft and very plastic material of not much use in engineering.
However, it can be alloyed with many elements. Alloys of iron and carbon are the most widely used in engineering.
The iron-carbon equilibrium diagram
A modern version of the iron-carbon equilibrium diagram is illustrated in Fig. 2.
The iron-carbon equilibrium diagram concerns transformations that occur in alloys having compositions from pure iron to cementite (6.67 per cent C). Point A (1539°C) on the diagram is the melting point of pure iron and point D (∼1550° C) is the melting point of iron carbide (cementite, Fe3C). Points N (1,401°C) and G (910° C) correspond to the allotropic transformations at α - iron into γ- iron. Point E shows the solubility limit of carbon in γ- iron at 1,130°C (2.0 percent).
The upper left-hand portion of the diagram represents the allotropic transformations γ⇌α (δ) at high temperatures. At temperatures on the line AB, crystals of the solid solution of carbon in α - iron ( δ solution) begin to precipitate from the liquid alloy. The line HJB represents peritectic transformation taking place at a constant temperature. The result of the peritectic reaction is the formation of the solid solution of carbon in γ - iron, i.e., austenite.
To simplify further study of the iron-carbon diagram we shall ignore this part of the diagram in the future since it is no practical importance. A simplified iron-carbon diagram is shown in Fig 3.
The alloys begin to solidify when they reach a temperature corresponding to the line ACD (liquidus) (Fig 3). Complete solidification corresponds to the solidus temperatures AECF.Austenite is precipitated from the liquid alloy along line AC and cementite along line CD. At point C with a temperature of 1,130° C and at a carbon content of 4.3 per cent austenite and cementite are simultaneously precipitated from the liquid alloy to form a eutectic called ledeburite.All alloys containing more than 2.0 per cent C (cast irons) and completely solidifying at 1,130° C (line ECF) form the eutectic (ledeburite).
A discussion of the primary solidification (transformation from the liquid to the solid state) of steels containing 0.75 per cent C and cast irons containing 3, 4.3, and 5.75 per cent C will clarify the interpretation of the diagram.
- Here the solid solution of carbon in γ - iron, austenite, begins to precipitate from the liquid alloy. The amount of austenite continuously increases as the temperature falls and its composition varies along the solidus (AE) while that of liquid phase varies along the liquids (AC). For example, at a temperature t.
- The composition of austenite corresponds to point a; that of the liquid phase is indicated by point b. This steel completely solidifies at temperature t.
- And will consist only of austenite. Steel of any composition will solidify in exactly the same manner and will consist of only austenite, at the moment when process has been completed.Cast iron containing 3.0 per cent C begins to solidify at temperature t.
- lying on the liquidus (AC). Here too austenite crystals precipitate from the liquid alloy in a continuously increasing amount as the temperature falls. The composition of the austenite and of the liquid phase may be determined at any temperature,t.
- By drawing a line through this temperature, parallel to the abscissa so that it intersects the liquids and the solidus, Point d indicates the austenite composition and point e, the composition of the liquid phase which is in equilibrium at temperature t.
- The proportion of the amounts of the liquid and solid phases at any temperature between the liquidus and solidus is determined by the lever rule. Thus, at the temperature t.
- The quantitative proportion of these phases is expressed by the ratio `frac{t_5d}{t_5e}`.Cast iron containing 3.0 per cent C completely solidifies at temperature t.
- (1130° C). At this temperature, the liquid phase, enriched in carbon up to 4.3 percent (the eutectic concentration), is finally solidified. From it, saturated austenite crystals (2.0 per cent C) precipitate,simultaneously with cementite to form the eutectic mixture, ledeburite.Therefore, directly following solidification,the cast iron will consist of primary austenite crystals and ledeburite, formed at 1130° C)(t).
- Cast iron of any composition between 2.0 to 4.3 per cent C (hypoeutectic cast irons) will solidify in exactly the same way as has been described above.
An alloy containing 4.3 per cent C (eutectic cast iron) differs from the previous alloys in that it solidifies at a constant temperature (point C at 1,130°C). Above this temperature, the alloy will be in a liquid state and when the temperature falls to 1130° C (point C), the alloy solidifies at this constant temperature forming ledeburite.
Solidification of the eutectic alloy at a constant temperature conforms to the phase rule. In a two component system containing three phases (liquid phase, austenite, and cementite) F = 2 +1 – 3 = 0, i.e., the system is nonvariant. All three phases have definite compositions (liquid phase = 4.3 per cent C, austenite – 2.0 per cent C, and Fe3 C – 6.67 per cent C) and the transformation temperature is constant at 1,130° C. A horizontal step on the cooling curves (Fig. 4) corresponds to the formation of the eutectic ledeburite).
Cast iron containing 4.3 per cent C will consist of only ledeburite after solidification. The solidification of hypereutectic cast irons (4.3 to 6.67 per cent C), as is evident form Fig. 4 begins along line CD by the precipitation of cementite (Fe3C) from the liquid alloy. A cast iron containing 5.75 per cent C, for example, begins to solidify at the temperature t 10.where cementite crystals (Fe3C) begin to precipitate from the liquid alloy. Since cementite is the phase richer in carbon, its precipitation will change the composition of the liquid alloy, upon a further fall in temperature,toward a reduction in carbon-content along the liquidus CD. At 1,130° C, the liquid alloy will reach the eutectic composition (4.3 per cent C) and will solidify at the (constant) temperature to form ledeburite. The completely solid alloy will consist of primary cementite crystals and ledeburite.All hypereutectic alloys will solidify in a similar manner.
Thus, all steels consist of austenite after the primary crystallization, while all cast irons consist of ledeburite and primary crystals of excess austenite (hypoeutectic cast irons containing from 2.0 to 4.3 per cent C) or cementite (hypereutectic cast irons containing from 4.3 to 6.67 per cent C).
We will next consider transformations known as secondary crystallisation which occur in the solid state. In the iron-carbon alloys, these are associated with the transformation of γ - iron to α - iron and the decomposition of austenite. The line GS in the equilibrium diagram (Fig. 3 and 5) indicates the beginning of austenite decomposition and the precipitation of ferrite from the austenite.
The critical points along the GS line are designated as Ac3 in heating and as Ar3 in cooling. The line SE indicates the temperatures at which austenite begins to decompose with the precipitation of excess carbon as cementite.This latter is called secondary or proeutectoid cementite to distinguish it from the cementite that precipitates from the liquid phase. Temperatures along the SE line are designated as Acm points. Points S, corresponding to 0.8 per sent C, shows the minimum temperature (723° C) at which austenite may exist in a state of equilibrium. At point S austenite decomposes with simultaneous precipitation of ferrite and cementite which form the eutectoid mixture known as pearlite.
The formation of pearlite proceeds at a constant temperature since, in the presence of three phases of constant composition (austenite, ferrite, and cementite), the number of degrees of freedom of the system will be zero (F = 2 + 1 = 3 0). Consequently, there is a horizontal step on the cooling curve at the pearlite formation temperature (Fig. 5).
In structure, pearlite consists of thin alternating plates of lamellae of cementite and ferrite called lamellar pearlite. As a result of a special heat treatment the so-called granular or divorced pearlite (cementite) may be formed. It consists of rounded globules of cementite in a ferrite field.
- The decomposition of austenite with the formation of pearlite corresponds to the line PSK (723° C) for all iron-carbon alloys. The temperature (critical point) at which pearlite is formed in cooling is designated as Ar1; the transformation of pearlite into austenite in heating occurs at a temperature designated as Ac1.
- Point P in Fig. 5 and 6 indicates the maximum solubility of carbon in α - iron at 723° C (0.025 percent). In the same way as the line SE running from point E indicates the reduction in the solubility of carbon in γ-iron (austenite) with a drop in temperature, the line PQ runs form P and indicates the reduction of the solubility of carbon in α - iron (ferrite) upon cooling the alloy.Therefore, alloys having a carbon content between points P and Q will consist of ferrite and excess (tertiary) cementite which usually precipitates along the boundaries of the ferrite grains (Fig. 6). The composition of the ferrite is indicated by the line PQ. After being completely cooled, alloys of a composition to the left of point Q will consist of only the solid solution of carbon in α -iron, i.e., of ferrite grains.
- We will next follow transformations that occur in steels containing from 0.025 to 0.8 per cent C (hypoeutectoid steels) when they are cooled from the solid solution (austenite) region.
- The diagram (Fig. 6) shows that no changes occur in the alloy until the temperature drops to the line GS. In this range, the alloys have a single-phase, austenite structure. Ferrite precipitates from the austenite at temperatures below line GS. Thus, a two- phase state exists below line GS: austenite (γ) and ferrite (α).
- Since the ferrite precipitating from the austenite upon further cooling contains very little carbon, the carbon concentration of the remaining austenite will continuously increase along the line GS.
- For example, in cooling a 0.2 per cent C steel, the austenite at temperature t2 will have a carbon content corresponding to point ‘a’ (Fig 7) while that of the ferrite will correspond to point c.Upon further cooling the carbon content of the austenite will increase and at a temperature of 723° C (Ac1), it will reach 0.8 per cent.
- Austenite of the eutectoid composition will decompose at the constant temperature of 723°C and pearlite will be formed.
- Thus, hypoeutectoid steels (containing less than 0.8 per cent C) will consist of ferrite and pearlite when they are completely cooled. The higher the carbon content of such steels, the more pearlite and the less ferrite they will contain.
- Other processes occur when steels containing more than 0.8 per cent C (hypereutectoid steels) are cooled. The line ES indicates the change in the solubility of carbon in austenite (Steel - iron) upon cooling. Only austenite exists above line ES. When the temperature drops to the line ES, the austenite is saturated with carbon which precipitates as cementite upon further cooling. Therefore, at temperatures below the line ES. a two-phase will exist, consisting of austenite and secondary or proeutectoid cementite Fe3CII (Fig. 7).
- The carbon content of the austenite is reduced upon the precipitation of cementite along the line ES. At a temperature of t4,the carbon content in the austenite will be 1.2 per cent (point b) when a hypereutectoid steel containing 1.35 per cent C is cooled.
- At the temperature 723° C (Ac1) the austenite will contain 0.8 per cent C and will decompose at this constant temperature into the ferrite-cementite eutectoid mixture which we call pearlite.
- Thus, completely cooled hypereutectoid steels (0.8 to 2.0 per cent C) will have a structure consisting of pearlite and excess (proeutectoid) cementite.
We may now consider the transformations that occur in cast irons. As has been mentioned above, cast irons contain ledeburite, after primary crystallisation, which is a eutectic consisting of saturated austenite (2.0 per cent C) and cementite. Hypoeutectic cast irons (2.0 to 4.3 per cent C) consist, in this state, of ledeburite and primary austenite crystals, containing 2.0 per cent C.Hypereutectic cast irons (4.3 to 6.67 per cent C) consist of primary cementite in addition to the ledeburite.
Upon further cooling, the solubility of carbon in the austenite is reduced (along line SE) and the primary crystals of the solid solution (austenite) will partly decompose, together with the austenite in the eutectic, with the precipitation of secondary cementite line crystals.The composition of the austenite will change along the line SE. When the line PSK (723° C) is reached, the
austenite, depleted of carbon to the eutectoid concentration (0.8 per cent C), decomposes into ferrite and cementite which form the eutectoid (pearlite).
Therefore, upon being completely cooled, hypoeutectic cast irons will have a structure consisting of pearlite, ledeburite (pearlite+ +cementite) and secondary cementite.
Eutectic cast iron consists of the eutectic – ledeburite-only. The ledeburite, in turn, consists of pearlite and cementite.
Hypereutectic cast irons consist of ledeburite and primary cementite, which is precipitated in the form of long plates or needles.
Uses and Applications
- Construction and Infrastructure: The iron and alloys, in particular, steel, are the basic materials in building and infrastructure sectors in many ways. These materials namely, steel, aluminum, copper, alloys, and others are in demand for construction of such structures as bridges, buildings, highways, railways and others because of their strength, durability and ease of use.
- Transportation: The iron, among other items is what makes the different transport modes be able to carry out their functions from road, sea, land and air. It is employed by car manufacturers. It help to produce engine components, chassis, and wheels and the outcome is the use of cars which are reliable and efficient.
- Machinery and Equipment: Modern manufacturing process engineered with Iron alloys is often utilized in the production of many household and industrial devices, including tools and machines. Iron-based materials such as workpiece, components offered to the industry with mechanical strength, wear resistance and machinability providing efficiency are used.
- Energy Generation and Transmission: Coal is used in the production of electrical generators, transformers, and power transmission infrastructure by iron. It is the fundamental substrate in the construction of transformers as well as firms with the operation of metal electrical energy generation, transmission and distribution.
- Consumer Goods: There are innumerable consumer products made of steel itself or its alloys, the likes of furniture, cutlery, cookware and appliances. They are used everyday in homes, offices, and commercial property, the aesthetic appeal, durability, and cheap price make them the top choice for these items.